Personalized Medicine in Pharmaceutical Manufacturing: Paving the Way for Tailored Therapies
Discover how personalized medicine is reshaping pharmaceutical manufacturing by tailoring treatments to individual needs. Learn about emerging technologies, regulatory insights, and real-world examples guiding the shift toward more precise, patient-centric therapies.
ThePharmaUniversity.org
2/13/20258 min read
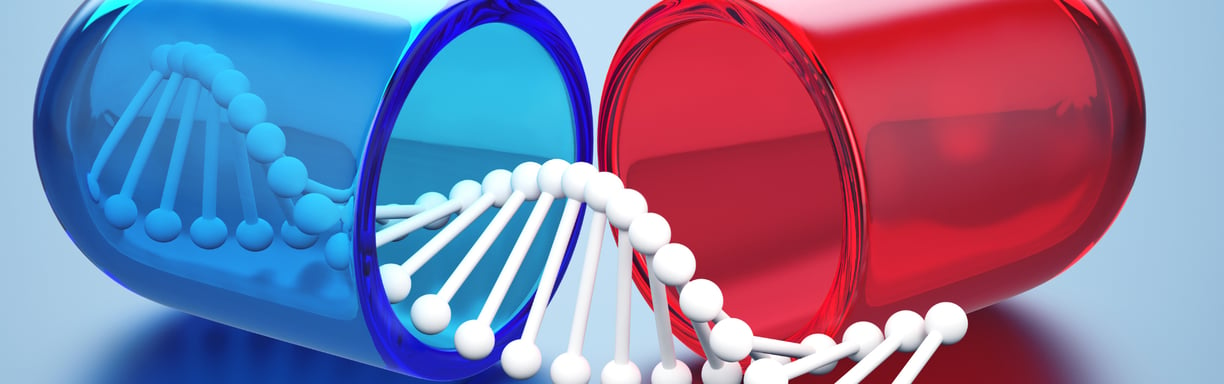
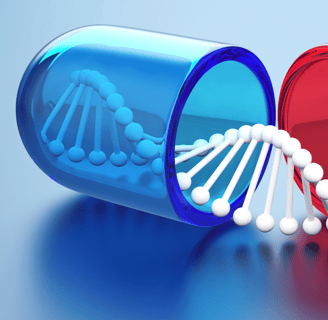
1. Introduction
Over the last decade, the concept of personalized medicine has captured the imagination of clinicians, researchers, and patients alike. Rooted in the understanding that each patient possesses a unique genetic and physiological profile, personalized medicine seeks to tailor therapeutic interventions to individuals rather than entire populations. This trend has profound implications for pharmaceutical manufacturing, reshaping not only how drugs are developed but also how they are produced, distributed, and monitored over time.
Historically, drug manufacturing has focused on large-scale, one-size-fits-all production, optimized for efficiency and cost-effectiveness. However, the emergence of personalized therapies—especially in areas like oncology, rare diseases, and genetic disorders—demands customized production processes that can meet individualized needs. Such therapies often require advanced biotechnological methods, tight regulatory oversight, and carefully orchestrated logistics to ensure that the right treatment reaches the right patient at the right time.
This comprehensive blog post explores the concept of personalized medicine in the context of pharmaceutical manufacturing, providing historical background, discussing regulatory frameworks, showcasing real-world case studies, and detailing the challenges and opportunities that lie ahead. While personalized medicine presents significant technical and economic hurdles, it also holds the promise of revolutionizing patient outcomes and ushering in a new era of precision health.
2. Background & Context
The Shift from Blockbuster Drugs to Targeted Therapies
In the 20th century, the pharmaceutical industry thrived on a blockbuster model—developing drugs like statins and anti-ulcer medications that could treat millions of patients globally. Manufacturing was geared toward mass production of uniform dosages, maximizing economies of scale. Although many of these drugs have been hugely successful, they cannot address the vast variability in individual patient responses.
As researchers gained deeper insights into genetics and molecular biology, they began to identify biomarkers—measurable indicators of disease states or treatment efficacy. This ushered in the age of targeted therapy, where drugs are designed to work for patients exhibiting specific genetic signatures. Notably, the Human Genome Project, completed in 2003, catalyzed discoveries in pharmacogenomics, revealing how genetic variations affect responses to certain drugs. This understanding laid the groundwork for truly personalized treatments.
Why Personalized Medicine Matters
Enhanced Efficacy: By focusing on the unique genetic or molecular profile of each patient, personalized therapies often achieve higher success rates than conventional treatments.
Reduced Adverse Effects: Knowing a patient’s genetic predisposition helps in selecting the right drug at the right dose, minimizing risks of severe side effects.
Targeted Populations: Instead of designing treatments for broad patient groups, manufacturers can tailor therapies to subsets of patients who are most likely to benefit, optimizing clinical outcomes and resource usage.
The rationale for personalized medicine is both clinical and economic: more precise therapies can reduce healthcare costs associated with trial-and-error approaches, decrease hospitalizations due to adverse drug reactions, and improve overall patient well-being. However, the manufacturing processes for these specialized treatments differ markedly from traditional, large-batch production lines.
3. Core Discussion
3.1. Defining Personalized Medicine
At its core, personalized medicine (also referred to as precision medicine) involves creating treatment plans based on individual characteristics—genetic, phenotypic, or molecular. Applications range from pharmacogenomic testing (e.g., checking if a patient’s genetic makeup makes them more likely to respond to a particular antidepressant) to cell and gene therapies that use a patient’s own cells or DNA to combat diseases like certain leukemias, lymphomas, or rare genetic disorders.
While personalized medicine’s scope is broad, this blog post focuses on how advanced therapeutics—particularly those requiring individualized manufacturing steps—impact pharmaceutical production.
3.2. Technological Foundations
Several technological developments enable the shift toward personalized treatments:
Genomic Sequencing: Rapid DNA sequencing helps identify genetic markers for diseases or drug responses. This data informs which patients might benefit from highly targeted therapies.
Bioprocessing Innovations: Techniques like cell culture bioreactors, viral vector production, and CRISPR gene editing facilitate complex biological manipulations at smaller scales.
Data Analytics & AI: Machine learning algorithms can parse enormous datasets to correlate genetic or molecular profiles with specific treatment outcomes. This automation shortens both drug discovery timelines and real-time manufacturing decisions (e.g., adjusting production parameters for a batch tailored to an individual).
Single-Use Technologies: Disposable bioreactors, tubing, and cell-culture systems reduce contamination risks and accelerate changeovers between personalized batches.
3.3. Impact on Manufacturing Efficiency, Quality, and Cost
Small-Batch or Single-Batch Production: Personalized treatments often involve individualized batches—especially in the case of cell therapies, where each batch is patient-specific. This creates a need for flexible production lines capable of rapid reconfiguration.
Strict Quality Control: Therapies derived from patient cells or tissues demand robust quality management to ensure safety. Small deviations in process parameters can significantly affect efficacy or safety. Real-time monitoring (e.g., using Process Analytical Technology, PAT) becomes crucial.
Higher Costs, but Potentially Lower Overall Healthcare Burden: While per-unit production costs may rise due to specialized manufacturing steps, effective personalized therapies can reduce hospital stays and subsequent treatments, possibly offsetting initial expenses. In some cases, a single administration of a gene therapy can lead to a lasting or curative benefit, significantly reducing long-term costs.
4. Regulatory & Compliance
Regulatory bodies such as the U.S. Food & Drug Administration (FDA) and the European Medicines Agency (EMA) recognize that personalized therapies require new frameworks for product approval, manufacturing guidelines, and post-market surveillance. Traditional regulatory pathways, designed around mass-produced drugs, may not always apply neatly to therapies made for one individual at a time.
Key considerations include:
cGMP Compliance for Biologics: The FDA outlines specific current Good Manufacturing Practices (cGMP) for biologically derived products, emphasizing contamination control, sterility, and traceability.
Advanced Therapy Medicinal Products (ATMPs): In Europe, gene and cell therapies are classified as ATMPs, with specialized guidelines focusing on clinical trials, manufacturing standards, and marketing authorization.
Real-Time Release & Testing: Personalized therapies often rely on real-time or near-real-time release tests because waiting for standard batch-release procedures isn’t feasible when the therapy is highly time-sensitive (e.g., autologous cell therapies).
Data Integrity & Patient Safety: Regulatory agencies also focus on electronic records and data management systems that meticulously track each batch to its patient, ensuring robust chain-of-identity (COI) and chain-of-custody (COC) controls.
For personalized medicine to thrive, early and continuous dialogue with regulators is critical. Companies that communicate their manufacturing approaches—particularly when they deviate from traditional, large-batch methods—can proactively address compliance, validation, and safety expectations.
5. Case Studies/Examples
5.1. CAR T-Cell Therapies
Chimeric Antigen Receptor (CAR) T-cell therapy is a powerful example of personalized medicine in oncology. The production process typically involves:
Leukapheresis: Collecting the patient’s T-cells from their bloodstream.
Genetic Modification: Inserting a gene encoding a specific receptor that allows these T-cells to recognize and attack cancer cells.
Cell Expansion: Growing the modified cells in bioreactors under tightly controlled conditions.
Patient Infusion: Returning the engineered T-cells to the patient, where they target cancer cells.
Approved CAR T therapies like Kymriah (Novartis) and Yescarta (Kite Pharma/Gilead) have shown remarkable success against certain leukemias and lymphomas. However, each batch is unique to the patient, requiring extremely careful handling, labeling, and time-sensitive production schedules. The cost, often exceeding USD $300,000 per treatment, highlights the complexity of this personalized approach. Nonetheless, for patients who have run out of conventional options, these therapies can offer life-saving possibilities.
5.2. Pharmacogenomics in Oncology
Another example involves pharmacogenomic testing for targeted cancer drugs—like trastuzumab (Herceptin) for HER2-positive breast cancer, or EGFR inhibitors for non-small cell lung cancer. While these therapies aren’t individually manufactured for each patient, their prescription is contingent upon molecular diagnostic tests confirming the presence of certain biomarkers. In this sense, the “manufacturing” adaptation lies in producing smaller, more targeted batches based on patient stratification rather than mass volume for an undifferentiated market.
6. Challenges & Considerations
6.1. Technical Complexities
Short Shelf Life: Many personalized therapies, especially cell-based products, have limited stability windows, necessitating ultra-cold storage or rapid delivery to clinical sites.
Customized Logistics: Each step—collection, modification, return—must be tracked meticulously. Delays or temperature excursions can render a therapy unusable.
Automation Gaps: While automation is well-established in conventional manufacturing, scaling it for personalized treatments is more challenging due to variable starting materials and smaller batch sizes.
6.2. Regulatory Hurdles
Evolving Guidelines: Personalized medicine is still relatively new, and regulatory agencies continually update guidance to reflect emerging technologies. Companies must remain agile.
Accelerated Approval Pathways: Some therapies qualify for breakthrough designations or fast-track approvals, but these pathways can impose additional post-market obligations.
Global Variation: Different regions have distinct requirements. Harmonizing manufacturing processes across multiple markets can be complex.
6.3. Economic and Access Barriers
High Production Costs: Individualized therapies often require specialized equipment and additional labor, driving up costs substantially compared to mass-produced drugs.
Reimbursement Dynamics: Insurance or national health services may be slow to cover high-cost personalized therapies, limiting patient access.
Scalability Limits: Meeting population-level demand with personalized approaches can strain manufacturing capacity, particularly for therapies that require extended lab work or custom viral vectors.
Potential Solutions
Modular and Flexible Facilities: Using single-use bioreactors and “clean-room pods” can help scale production capacity and rapidly adapt to different therapies.
Investments in Process Automation: Automating critical steps—cell isolation, gene transfer, packaging—can mitigate human error and help reduce costs over time.
Innovative Payment Models: Health systems and manufacturers might explore outcome-based payment models, reducing payer risk if a therapy fails to meet clinical goals.
Early Stakeholder Engagement: Collaborating with regulators, patients, and payers from the start can accelerate approvals and improve market access.
7. Future Outlook
7.1. Manufacturing Innovations
As personalized medicine becomes more mainstream, new technologies will emerge to streamline and economize production:
Microfluidic Platforms: Tiny chips could automate cell processing steps, reducing manual labor and contamination risks.
AI-Driven Process Optimization: Advanced algorithms can tailor each production run to the patient’s unique cell characteristics, adjusting parameters like growth media composition or temperature in real time.
Distributed Manufacturing: Localized manufacturing centers or even hospital-based “mini factories” could expedite turnaround times for autologous therapies, bypassing lengthy shipping logistics.
Smart Supply Chains: Blockchain and IoT solutions might track each step, ensuring chain-of-identity from collection to infusion and preventing mix-ups.
7.2. Broader Impact on Healthcare
Widespread adoption of personalized medicine could reshape entire healthcare systems:
Preventive Medicine: Genomic testing may become routine, identifying individuals at risk for specific conditions. Early interventions, including lifestyle modifications or prophylactic treatments, could reduce overall healthcare costs.
Collaborative Ecosystems: Pharmaceutical companies, diagnostic labs, and healthcare providers will likely form close partnerships to coordinate the many moving parts—data collection, therapy design, logistics, and payment.
Ethical & Social Considerations: Access, equity, and privacy remain hot-button issues. As genetic data becomes integral to treatment, ensuring secure data handling and fair treatment access will be paramount.
8. Conclusion
Personalized medicine represents a paradigm shift in how we discover, develop, and manufacture therapies. It transcends the traditional boundaries of large-scale production by embracing individual patient differences, enabling potent new treatments in oncology, rare diseases, and beyond. From CAR T-cell therapies that genetically re-engineer a patient’s immune cells, to targeted cancer drugs guided by precise molecular diagnostics, the pharmaceutical industry is witnessing a revolution in which data, technology, and patient specificity converge.
Yet, this revolution does not come without its share of challenges. The shift toward flexible, small-batch production raises questions about cost-effectiveness, supply chain complexities, and regulatory compliance. Smaller companies may struggle to afford the advanced infrastructure required, while established players face the hurdle of integrating new processes into their existing manufacturing ecosystems. Regulatory agencies, in turn, need to refine guidelines to keep pace with the rapid proliferation of new therapies.
Despite these obstacles, the future outlook is undeniably promising. Technological innovations—from AI-driven process controls to single-use bioreactors—are steadily making personalized manufacturing more feasible and efficient. As the industry continues to collaborate with regulators, payers, and patients, personalized medicine could well become a cornerstone of modern healthcare, offering transformative benefits for millions of people who currently have limited or ineffective treatment options.
References
FDA Guidance on Gene Therapy Manufacturing:
https://www.fda.gov/vaccines-blood-biologics/cellular-gene-therapy-productsEMA Guidelines on Advanced Therapy Medicinal Products:
https://www.ema.europa.eu/en/human-regulatory/overview/advanced-therapy-medicinal-products-overviewNovartis CAR T-Cell Therapy (Kymriah):
https://www.novartis.com/our-science/novartis-cell-and-gene-therapy/kymriahGilead/Kite CAR T-Cell Therapy (Yescarta):
https://www.kitepharma.com/our-science/yescartaPharmacogenomics Research Network:
https://www.genome.gov/Funded-Programs-Projects/Pharmacogenomics-Research-Network
The Pharma University
Empowering professionals in pharmaceutical manufacturing with expert resources, training, and insights to ensure GMP compliance and drive innovation.
Subscribe to The Pharma University and unlock exclusive insights, resources, and updates to advance your pharmaceutical career. Join our community of industry professionals today!
© 2025 ThePharmaUniversity.org.
All Rights Reserved.
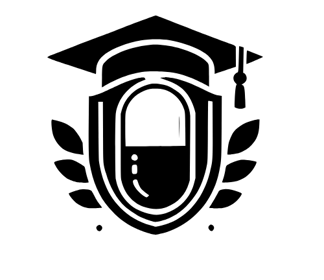
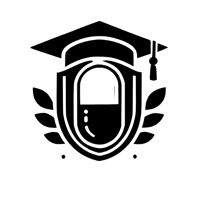